Magnetism: Magnetic Forces and Magnetic Fields
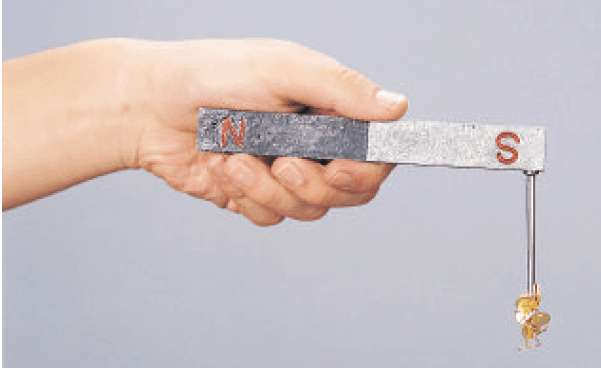
If you have ever used a compass or picked up tacks or paper clips with a magnet, you have observed some effects of magnetism. You even might have made an electromagnet by winding wire around a nail and connecting it to a battery. The properties of magnets become most obvious when you experiment with two of them. To enhance your study of magnetism, you can experiment with magnets, such as those shown in the Figure.
Digital lesson: How Do We Use Electricity?
|
Digital lesson: Magnets And Magnetism
|
Digital lesson: Electromagnetism
|
---|
Magnets
You probably have observed a magnet attract paper clips, nails, and other objects made of iron. Any magnet, whether it is in the shape of a bar or a horseshoe, has two ends or faces, called poles, which is where the magnetic effect is strongest. If a bar magnet is suspended from a fine thread, it is found that one pole of the magnet will always point toward the north. It is not known for sure when this fact was discovered, but it is known that the Chinese were making use of it as an aid to navigation by the eleventh century and perhaps earlier.
This is the principle of a compass. A compass needle is simply a bar magnet which is supported at its center of gravity so that it can rotate freely. The pole of a freely suspended magnet that points toward geographic north is called the north pole of the magnet. The other pole points toward the south and is called the south pole.
It is a familiar observation that when two magnets are brought near one another, each exerts a force on the other. The force can be either attractive or repulsive and can be felt even when the magnets don’t touch. If the north pole of one bar magnet is brought near the north pole of a second magnet, the force is repulsive. Similarly, if the south poles are brought close, the force is repulsive. But when the north pole of one magnet is brought near the south pole of another magnet, the force is attractive. These results are reminiscent of the forces between electric charges: like poles repel, and unlike poles attract. But do not confuse magnetic poles with electric charge. They are very different. One important difference is that a positive or negative electric charge can easily be isolated. But an isolated single magnetic pole has never been observed. If a bar magnet is cut in half, you do not obtain isolated north and south poles. Instead, two new magnets are produced, each with north (N) and south (S) poles. If the cutting operation is repeated, more magnets are produced, each with a north and a south pole. Physicists have searched for isolated single magnetic poles (monopoles), but no magnetic monopole has ever been observed.
Digital lesson: What Are Magnets?
|
Digital lab: How Strong Is a Magnet?
|
---|
Magnetic Fields and Magnetic Field Lines
Magnets can exert forces on each other. The magnetic forces between north and south poles have the property that like poles repel each other, and unlike poles attract. This behavior is similar to that of like and unlike electric charges. However, there is a significant difference between magnetic poles and electric charges. It is possible to separate positive from negative electric charges and produce isolated. charges of either kind. In contrast, no one has found a magnetic monopole (an isolated north or south pole). Any attempt to separate north and south poles by cutting a bar magnet in half fails, because each piece becomes a smaller magnet with its own north and south poles.
Ferromagnets and Electromagnets
Besides iron, a few other materials, such as cobalt, nickel, gadolinium, and some of their oxides and alloys, show strong magnetic effects. They are said to be ferromagnetic (from the Latin word ferrum for iron). Other materials show some slight magnetic effect, but it is very weak and can be detected only with delicate instruments. We will look in more detail at ferromagnetism.
The Force on a Moving Charge in a Magnetic Field
We saw that when a charge is placed in an electric field, it experiences an electric force. And when a charge is placed in a magnetic field, it also experiences a force, provided that certain conditions are met, as we will see. The magnetic force, like all the forces we have studied (e.g., the gravitational, elastic, and electric forces), may contribute to the net force that causes an object to accelerate. Thus, when present, the magnetic force must be included in Newton's second law.
The following two conditions must be met for a charge to experience a magnetic force when placed in a magnetic field:
1. The charge must be moving, because no magnetic force acts on a stationary charge.
2. The velocity of the moving charge must have a component that is perpendicular to the direction of the magnetic field.
The Motion of a Charged Particle in a Magnetic Field
The motion of a charged particle in an electric field is noticeably different from the motion in a magnetic field. For example, a positive charge moving between the plates of a parallel plate capacitor. Initially, the charge is moving perpendicular to the direction of the electric field. Since the direction of the electric force on a positive charge is in the same direction as the electric field, the particle is deflected sideways. If the same particle traveling initially at right angles to a magnetic field. An application of RHR-1 shows that when the charge enters the field, the charge is deflected upward (not sideways) by the magnetic force. As the charge moves upward, the direction of the magnetic force changes, always remaining perpendicular to both the magnetic field and the velocity.
A charged particle traveling in a magnetic field experiences a magnetic force that is always perpendicular to the field. In contrast, the force applied by an electric field is always parallel (or antiparallel) to the field direction. Because of the difference in the way that electric and magnetic fields exert forces, the work done on a charged particle by each field is different.
The Mass Spectrometer
Physicists use mass spectrometers for determining the relative masses and abundances of isotopes. Chemists use these instruments to help identify unknown molecules produced in chemical reactions. Mass spectrometers are also used during surgery, where they give the anesthesiologist information on the gases, including the anesthetic, in the patient's lungs.
The Hall Effect
When a current-carrying conductor is held fixed in a magnetic field, the field exerts a sideways force on the charges moving in the conductor. For example, if electrons move to the right in the rectangular conductor, the inward magnetic field will exert a downward force on the electrons of magnitude $F= e v B$ where $v$ is the drift velocity of the electrons. Thus the electrons will tend to move nearer to one side than other side , causing a potential difference between the two sides of the conductor. This potential difference builds up until the electric field that it produces exerts a force on the moving charges that is equal and opposite to the magnetic force .
This is the Hall effect, named after E. H. Hall who discovered it in 1879. The difference of potential produced is called the Hall emf. Its magnitude is $V_{Hall}= E_H d = \dfrac{ F}{e} d = v B d$ , where $d$ is the width of the conductor.
Animated Physics: Magnetic Force
|
Interactive Demonstration: Particle in a Magnetic Field
|
---|---|
Digital Investigation: charge in a magnetic field
|
Digital simulation: Motion of a Charge in Magnetic and Elecytric Fields
|
Digital figure: charged particle moving in a magnetic field
|
Digital Interactives: Magnetic Fields
|
The Force on a Current-Carrying Conductor in a Magnetic Field
As we have seen, a charge moving through a magnetic field can experience a magnetic force. Since an electric current is a collection of moving charges, a current in the presence of a magnetic field can also experience a magnetic force. For instance, a current-carrying wire is placed between the poles of a magnet. When the direction of the current $I$ is perpendicular to the magnetic field, the moving charges experience a magnetic force that pushes the wire to the right. The direction of the force is determined in the usual manner by using RHR-1, with the minor modification that the direction of the velocity of a positive charge is replaced by the direction of the conventional current $I$. If the current were reversed by switching the leads to the battery, the direction of the force would be reversed, and the wire would be pushed to the left.
The Torque on a Current-Carrying Loop in a Magnetic Field: Motors and Meters
We have seen that a current-carrying wire can experience a force when placed in a magnetic field. If a loop of wire is suspended properly in a magnetic field, the magnetic force produces a torque that tends to rotate the loop. This torque is responsible for the operation of a widely used type of electric motor.
Interactive Demonstration: Force on a Current-Carrying Conductor
|
Digital figure: wire carries a current in a magnetic field
|
---|
Magnetic Fields Produced by Currents
We have seen that a current-carrying wire can experience a magnetic force when placed in a magnetic field that is produced by an external source, such as a permanent magnet. A current-carrying wire also produces a magnetic field of its own, as we will see in this section. Hans Christian Oersted (1777-1851) first discovered this effect in 1820 when he observed that a current-carrying wire influences the orientation of a nearby compass needle. The compass needle aligns itself with the net magnetic field produced by the current and the magnetic field of the earth. Oersted's discovery, which linked the motion of electric charges with the creation of a magnetic field, marked the beginning of an important discipline called electromagnetism.
Ampere’s Law
We have seen that an electric current creates a magnetic field. However, the magnitude and direction of the field at any point in space depends on the specific geometry of the wire carrying the current. For instance, distinctly different magnetic fields surround a long, straight wire, a circular loop of wire, and a solenoid. Although different, each of these fields can be obtained from a general law known as Ampere's law, which is valid for a wire of any geometrical shape. Ampere's law specifies the relationship between an electric current and the magnetic field that it creates.
Concept Map: Magnetic Fields
|
Digital simulation: Magnetic Filed Produced by Long Straight Current-Carrying Wire
|
Digital simulation: Magnetic Field Produced by a Current Loop
|
Digital simulation: Magnetic Field Produced by a Solenoid
|
---|
You don`t have permission to comment here!
Report